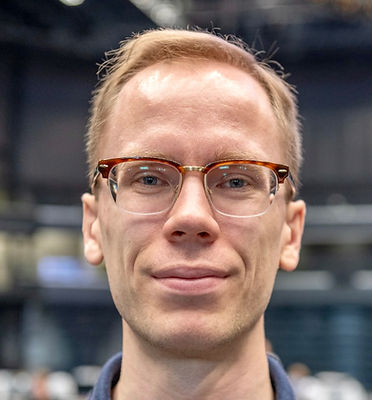
DR. ANTON FRISK KOCKUM
I'm a theoretical physicist working on various aspects of quantum optics and quantum information, including circuit quantum electrodynamics, ultrastrong light-matter coupling, and quantum acoustics. I received my Ph.D. degree in physics from Chalmers University of Technology in 2014. I then moved to Japan to be a postdoctoral researcher in the group of Franco Nori at RIKEN, where I was supported for two years through a JSPS Fellowship. In 2018, I moved back to Sweden, taking up a permanent position as a researcher at the Wallenberg Centre for Quantum Technology (WACQT), at Chalmers University of Technology. From June 2020 to November 2022, I was also the scientific coordinator of WACQT. In October 2022, I was promoted to senior researcher and in May 2024 I became associate professor.
​
SOME RESEARCH HIGHLIGHTS

Waveguide quantum electrodynamics with giant superconducting artificial atoms
Bharath Kannan, Max J. Ruckriegel, Daniel L. Campbell, Anton Frisk Kockum, Jochen Braumüller, David K. Kim, Morten Kjaergaard, Philip Krantz, Alexander Melville, Bethany M. Niedzielski, Antti Vepsäläinen, Roni Winik, Jonilyn L. Yoder, Franco Nori, Terry P. Orlando, Simon Gustavsson, and William D. Oliver
arXiv:1912.12233
​
Models of light-matter interactions typically invoke the dipole approximation, within which atoms are treated as point-like objects when compared to the wavelength of the electromagnetic modes that they interact with. However, when the ratio between the size of the atom and the mode wavelength is increased, the dipole approximation no longer holds and the atom is referred to as a "giant atom". Thus far, experimental studies with solid-state devices in the giant-atom regime have been limited to superconducting qubits that couple to short-wavelength surface acoustic waves, only probing the properties of the atom at a single frequency. Here we employ an alternative architecture that realizes a giant atom by coupling small atoms to a waveguide at multiple, but well separated, discrete locations. Our realization of giant atoms enables tunable atom-waveguide couplings with large on-off ratios and a coupling spectrum that can be engineered by device design. We also demonstrate decoherence-free interactions between multiple giant atoms that are mediated by the quasi-continuous spectrum of modes in the waveguide-- an effect that is not possible to achieve with small atoms. These features allow qubits in this architecture to switch between protected and emissive configurations in situ while retaining qubit-qubit interactions, opening new possibilities for high-fidelity quantum simulations and non-classical itinerant photon generation.

Fast multi-qubit gates through simultaneous two-qubit gates
Xiu Gu, Jorge Fernández-Pendás, Pontus Vikstål, Tahereh Abad, Christopher Warren, Andreas Bengtsson, Giovanna Tancredi, Vitaly Shumeiko, Jonas Bylander, Göran Johansson, and Anton Frisk Kockum
arXiv:2108.11358
​
Near-term quantum computers are limited by the decoherence of qubits to only being able to run low-depth quantum circuits with acceptable fidelity. This severely restricts what quantum algorithms can be compiled and implemented on such devices. One way to overcome these limitations is to expand the available gate set from single- and two-qubit gates to multiqubit gates, which entangle three or more qubits in a single step. Here, we show that such multiqubit gates can be realized by the simultaneous application of multiple two-qubit gates to a group of qubits where at least one qubit is involved in two or more of the two-qubit gates. Multiqubit gates implemented in this way are as fast as, or sometimes even faster than, the constituent two-qubit gates. Furthermore, these multiqubit gates do not require any modification of the quantum processor, but are ready to be used in current quantum-computing platforms. We demonstrate this idea for two specific cases: simultaneous controlled-Z gates and simultaneous iswap gates. We show how the resulting multiqubit gates relate to other well-known multiqubit gates and demonstrate through numerical simulations that they would work well in available quantum hardware, reaching gate fidelities well above 99%. We also present schemes for using these simultaneous two-qubit gates to swiftly create large entangled states like Dicke and Greenberger-Horne-Zeilinger states.